ACORN is dedicated to advancing the understanding of glaucoma and visual neurodegenerative diseases through four key pillars:
I. Understanding Disease Mechanism: Our mission is to delve into the intricate mechanisms underlying the onset and progression of glaucoma. Our focus is to better understand the disease mechanism which might lead to identifying new treatment targets.
II. Detection, Monitoring, and Prediction of Disease: We strive to pioneer methods for early detection, sensitive monitoring, and precise prediction of disease progression. Our goal is to prevent vision loss and blindness by identifying and addressing disease trajectory at their earliest stages.
III. Advancement of Imaging Technology: Early detection is paramount, as timely intervention can make a significant difference in preserving functional vision. We push the boundaries of imaging technologies, seeking to provide clearer and more comprehensive insights into the progression of glaucoma and other visual neurodegenerative disease. By exploring novel technologies and parameters, we strive to advance the way we visualize and understand these diseases.
IV. Innovative Analytical Methods: Our focus extends to developing sophisticated analytical tools, including statistical and artificial intelligence (AI) algorithms. These tools enable extraction of valuable information from complex datasets, furthering understanding of disease mechanisms and improving prediction.
Our unique multidisciplinary team, comprised of clinicians, engineers, and data scientists, allows us to tackle several facets of the disease from different perspectives and levels of expertise. Along with our ongoing collaboration with academic leaders ensure that we are at the forefront of discovery and innovation.
Our lab and researchers are in the forefront of development, clinical implementation and evaluation of cutting-edge ophthalmic imaging technologies. This work started more than two decades ago with groundbreaking research using optical coherence tomography (OCT) and confocal scanning laser ophthalmoscopy (CSLO).
Recent research focus of the lab includes the visible-light (vis-OCT), an innovative iteration of OCT capable of capturing unprecedentedly high-resolution images of the retina [1]. These technological enhancements open doors to novel diagnostic and monitoring approaches.
Recognizing that glaucoma often originates from changes in the anterior segment angle and the outflow pathway, we developed novel methods to visualize and quantify swept source OCT (SS-OCT) images. Our approach involves leveraging episcleral vasculature OCT angiography to elucidate the intricate relationship between anterior segment vasculature and aqueous outflow facility.
By incorporating all clinically available and novel protype technologies, ranging from adaptive optics (AO) to scanning laser ophthalmoscopy (SLO), and OCT, we are pushing the boundaries of imaging to achieve cellular-level visualization of retinal microstructures, enabling investigation at cellular level with unprecedented clarity [2].
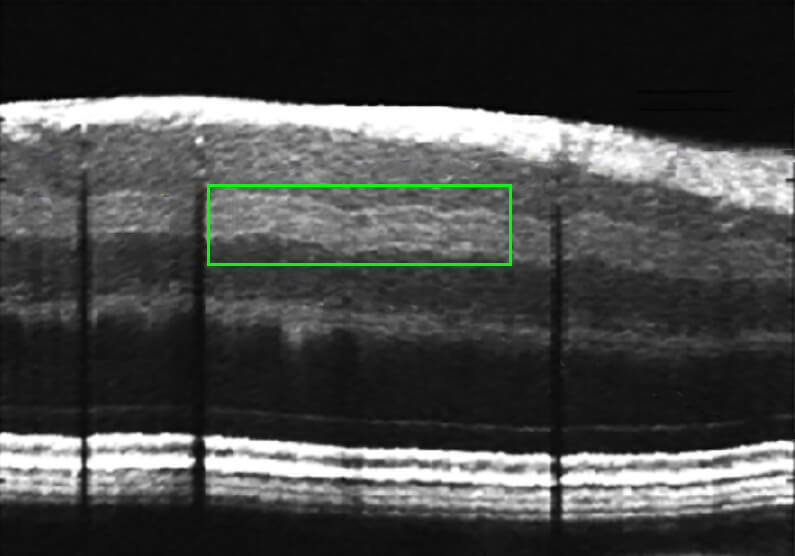
Visualization of previously unseen retinal inner plexiform layer (IPL) sublayers associated with thinning of retina in glaucoma observed with Visible Light OCT [8].
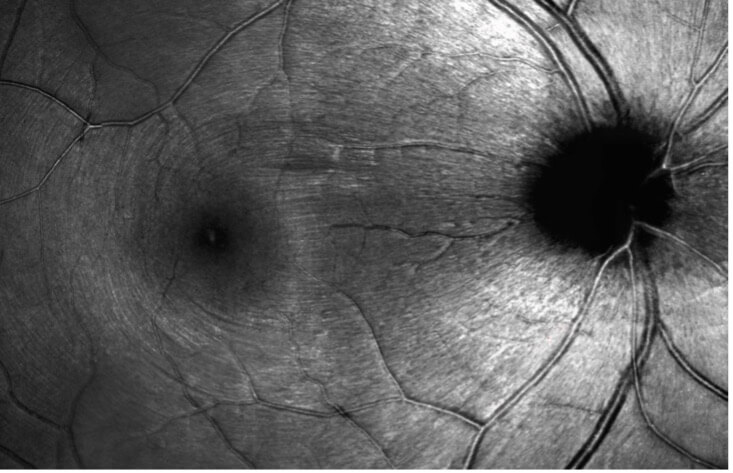
Adaptive optics SLO imaging of retinal nerve fiber bundles of the retina in a healthy eye.
A significant aspect of our research is dedicated to unraveling the intricate workings of glaucoma. We are using advance image processing methods developed in the lab to investigate the in-vivo, three-dimensional structure of primary targets of initial glaucoma damage in the optic nerve and the Lamina cribrosa (LC) within it [3,4]. We reported morphological features within the LC in glaucomatous eyes that are distinct from those in healthy eyes which might be associated with the disease mechanism[5,6].
Using robust animal models, we have demonstrated that morphological and mechanical changes within the LC volume occur in response to acute intraocular and intracranial pressure modulations [7]. Elevated pressure exerted on the LC is causing tension on the passing retinal ganglion cells axons, ultimately leading to the demise of these cells. We also reported larger variation across eyes in the structural and the toursousitiy response to acute IOP modulations [8,9]. This variability in LC characteristics might explain the inter-individual differences in ocular glaucomatous damage and potentially serve as a biomarker for the disease and novel treatment target.
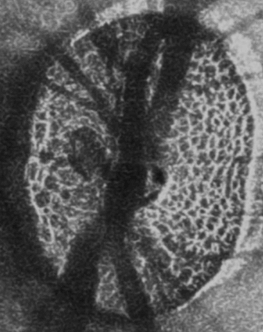
Visualization of lamina cribrosa in vivo.
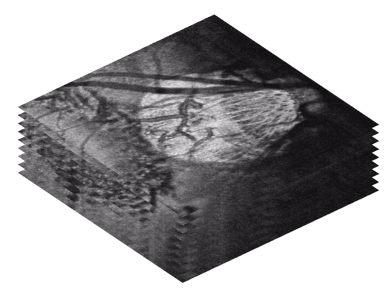
Pore tortuosity showing path of the retinal ganglion cells axons as they pass through the LC. [6]
3. Repeatability of in vivo 3D lamina cribrosa microarchitecture using adaptive optics spectral domain optical coherence tomography
4. Reproducibility of in-vivo OCT measured three-dimensional human lamina cribrosa microarchitecture
5. In Vivo Lamina Cribrosa Micro-Architecture in Healthy and Glaucomatous Eyes as Assessed by Optical Coherence Tomography
6. Tortuous Pore Path Through the Glaucomatous Lamina Cribrosa.
7. 3D Microstructure of the Healthy Non-Human Primate Lamina Cribrosa by Optical Coherence Tomography Imaging.
8. In-vivo effects of intraocular and intracranial pressures on the lamina cribrosa microstructure.
9. Under Pressure: Lamina Cribrosa Pore Path Tortuosity in Response to Acute Pressure Modulation.
Glaucoma is a highly variable disease, posing challenges in predicting its trajectory and preventing irreversible vision loss. Early detection is paramount, and our group has made significant contributions to elucidating key aspects of glaucoma diagnosis and progression monitoring.
We reported on the relationship between ocular structure and function and the deflection point at which structural changes detected by OCT begin to impact functional vision [10]. We have shown that progression paths of glaucoma vary depending on disease severity, and that longitudinal changes in peripapillary atrophy area measured by OCT can be an indicator of structural and functional glaucoma progression [11].
Our group explores novel functional testing methodologies, including virtual reality oculokinetic perimetry testing, aimed at refining functional measurements of vision [12]. These advancements hold promise for improving clinical outcomes and patient care.
10. Retinal nerve fibre layer and visual function loss in glaucoma: the tipping point.
11. Peripapillary Atrophy Area as an Indicator of Glaucomatous Structural and Functional Progression.
12. Virtual reality oculokinetic perimetry test reproducibility and relationship to convential perimetry and OCT.
Harnessing the power of artificial intelligence (AI) deep learning algorithms for disease detection, our group has leveraged OCT imaging data to predict disease progression with high precision. Our studies have demonstrated the ability to predict spatially resolved functional loss [13]. These AI-derived features hold promise for assisting healthcare providers in clinical decision-making, especially in cases where functional testing lacks accuracy or sensitivity.
The integration of artificial intelligence and advanced analytic tools have profound implications for diagnosis and treatment. We've pioneered the enhancement of retinal OCT images through deep learning techniques, resulting in high-definition representations that facilitate precise diagnosis and treatment planning [14]. Furthermore, we've developed feature-agnostic methodologies for detecting glaucoma in OCT volumes, enhancing diagnostic sensitivity and specificity [15].
We have demonstrated improvements to disease prognostication by integrating multimodal temporal data, including OCT volumes, to enable the development of predictive models for disease trajectory optimization [16]. Additionally, we're developing tools like the 2D-Hidden-Markov Model and LIMBARE to provide robust estimation methods for longitudinal ophthalmic studies, yielding insights into disease dynamics and treatment response [17,18].
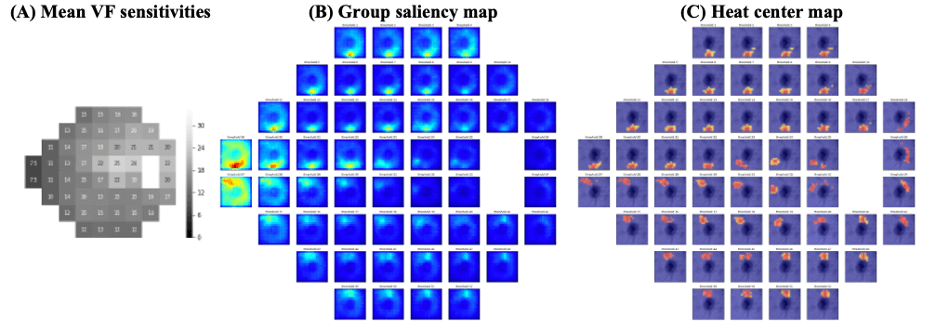
Artificial Intelligence for predicting visual field defects at pointwise level can be made using OCT volumes. [13]
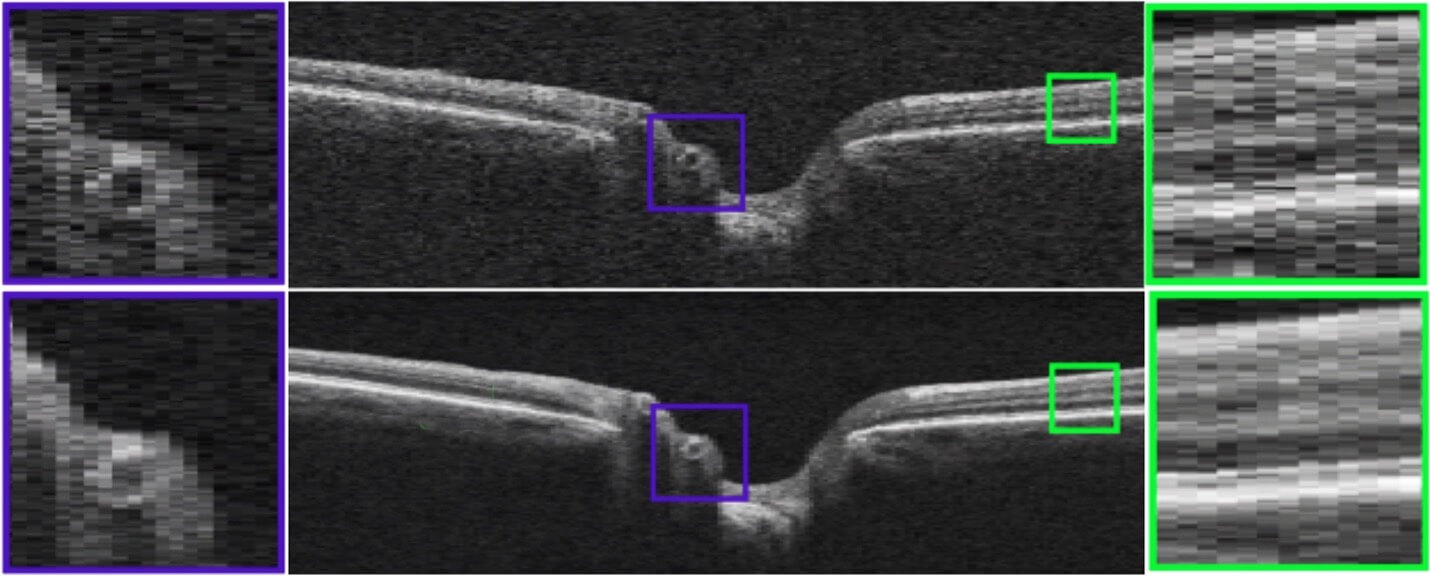
Example of AI-based enhancement of OCT image quality. Top, original OCT. Bottom, AI enhanced image. [14]
13. Segmentation-Free OCT-Volume-Based Deep Learning Model Improves Pointwise Visual Field Sensitivity Estimation.
14. Retinal optical coherence tomography image enhancement via deep learning.
15. A feature agnostic approach for glaucoma detection in OCT volumes.
16. Forecasting Retinal Nerve Fiber Layer Thickness from Multimodal Temporal Data Incorporating OCT Volumes.
17. Clinical Prediction Performance of Glaucoma Progression Using a 2-Dimensional Continuous-Time Hidden Markov Model with Structural and Functional Measurements.
18. LIMBARE: An Advanced Linear Mixed-Effects Breakpoint Analysis With Robust Estimation Method With Applications to Longitudinal Ophthalmic Studies.
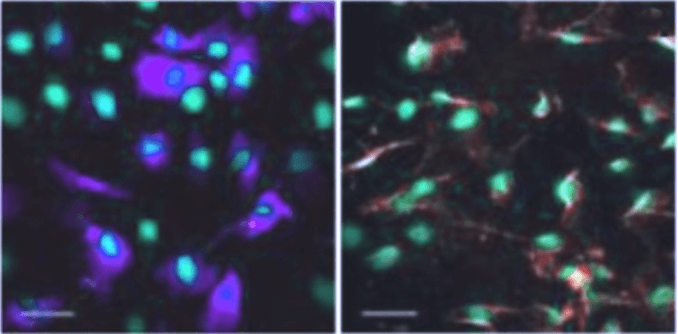
Stem cell regeneration of Trabecular Meshwork study. [19]
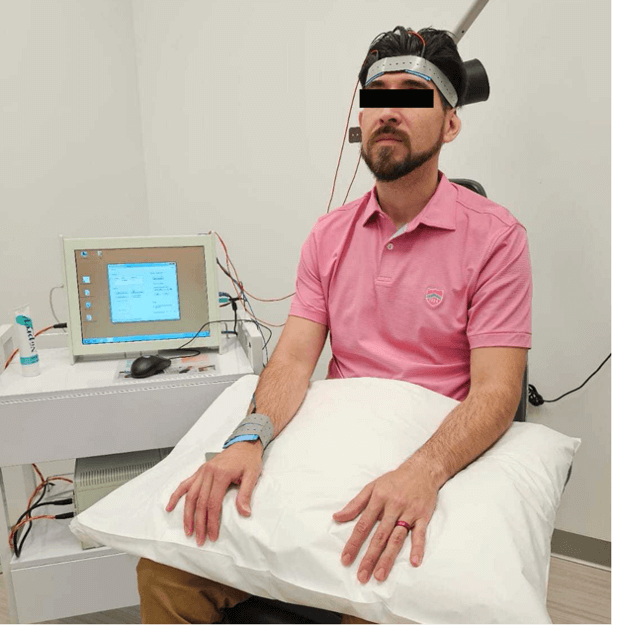
rtACS electrostimulation therapy subject. [20]
Our research group is exploring innovative therapies for neuroenhancement and regenerative medicine in ocular diseases. We are actively exploring the therapeutic potential of regeneration of the trabecular meshwork (TM), a crucial component involved in maintaining normal ocular outflow, through the use of stem cells. We have demonstrated in rodent models that transplantation of adipose-derived mesenchymal stem cells can differentiate into TM-like cells, integrate into TM tissue, and regulate intraocular pressure [19].
Our group is also studying the potential benefits of electrical stimulation therapy, which has shown efficacy in addressing functional deficits associated with various vision and neurodegenerative disorders. Currently, we are conducting a double-masked randomized clinical trial to evaluate the effectiveness of repetitive transorbital alternating current stimulation (rtACS), a form of electrical neurostimulation that delivers a gentle periorbital electric current [20].
We reported that race and ethnicity impact the likelihood of glaucoma and its progression [21]. However, less is understood about the role of social biases and the non-genetic role of race in health care management and disease progression. Our group have shown that Black and Asian subjects with glaucoma receive fewer visual field tests per visit compared to White subjects even when considering socioeconomic disadvantage and disease severity [22]. We understand the importance of minimizing the effect of biases in treatment and are actively developing methods for improving analysis, such as handling data imbalance often observed when evaluating disparity with marked differences between the tested groups by leveraging advanced transfer learning [23].